Selecting Casing Grade for a Deep Sour HPHT Well (27,000 ft, Gulf Coast)
- Samuel Ighalo
- Mar 25
- 21 min read
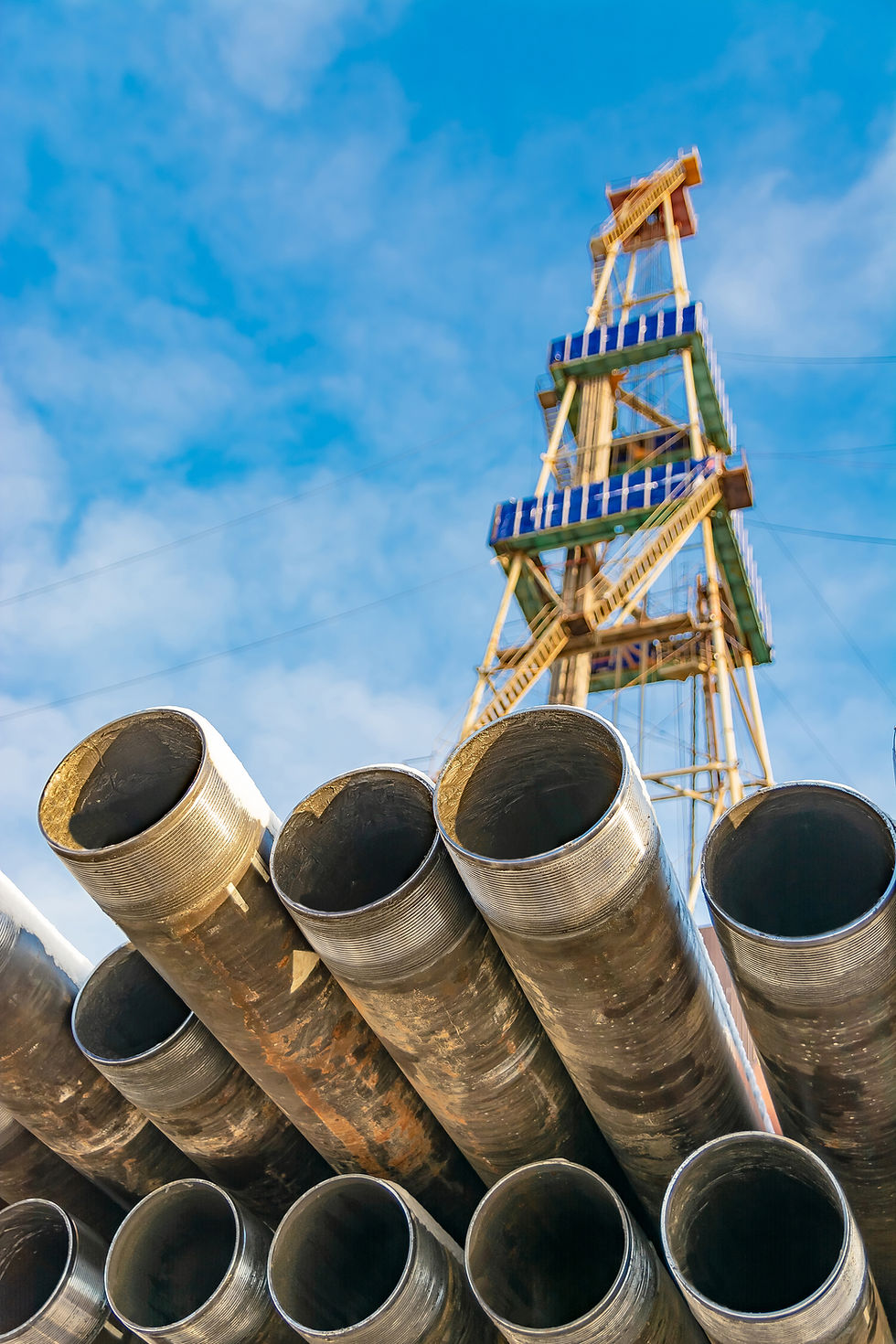
Introduction
Drilling a 27,000-ft exploratory well in Louisiana’s Gulf Coast with high hydrogen sulfide (H₂S) and carbon dioxide (CO₂) poses extreme demands on casing material selection. Such wells qualify as HPHT (High-Pressure, High-Temperature) by API definitions – pressures >15,000 psi and temperatures >350 °F and also as sour service environments due to the presence of “wet” H₂S. Sour HPHT conditions can cause rapid corrosion and catastrophic sulfide stress cracking (SSC) or hydrogen-induced cracking (HIC) in standard casing steels.
Selecting the right casing grade is therefore a critical engineering decision balancing mechanical strength, SSC/HIC resistance, CO₂ corrosion resistance, cost, and supply chain considerations. This blog will review the engineering criteria and industry standards (API 5CT, NACE MR0175/ISO 15156, API Technical Reports) for material selection in deep sour wells, and compare common casing grades – from carbon steels (L80, C95, T95, P110, Q125) to corrosion-resistant alloys (13Cr, duplex, nickel-based) – including their performance trade-offs, cost, and implications for cementing and completion strategies.
Challenges in a Sour HPHT Environment
Sour Gas Exposure (H₂S): In a sour environment, even trace H₂S in the presence of water can embrittle high-strength steels. Wells are generally considered “sour” if the H₂S partial pressure exceeds 0.05 psi (0.3 kPa). In such conditions, standard high-strength casing grades can suffer SSC – a hydrogen embrittlement mechanism that leads to brittle cracking under tensile stress. The risk of SSC increases with higher steel hardness and strength; thus, as steel yield strength rises, its susceptibility to sulfide cracking also increases. H₂S can also cause hydrogen-induced cracking (HIC) in lower-strength steels, where hydrogen blisters form and coalesce into planar cracks within the metal. These cracking mechanisms are most acute at ambient-to-moderate temperatures (where hydrogen diffusion is slow), meaning even during well shut-in or cool-down, the casing can be at risk. For a deep Louisiana well that encounters sour gas, SSC and HIC resistance is a foremost selection criterion to ensure well integrity.
CO₂ Corrosiveness: CO₂ (acid gas) produces carbonic acid when dissolved in water, leading to sweet corrosion of steel. CO₂ corrosion causes uniform thinning and can accelerate at high temperatures common in deep wells. In combination with H₂S, CO₂ can aggravate pitting and localized attack. Carbon steels without mitigation can corrode rapidly in high-CO₂ fluids, threatening casing integrity. Adding chromium significantly improves resistance – for example, 13Cr stainless (L80-13Cr) is widely used for CO₂-rich wells because its chromium oxide film combats carbonic acid attack. However, 13Cr has limits in sour service (it too can crack if H₂S is above minimal levels). Thus, material selection must account for CO₂ corrosion rates and possibly utilize corrosion-resistant alloys (CRAs) or inhibitors to handle long-term exposure.
High Pressure & Temperature: A 27,000-ft well likely exhibits extreme pressures and temperatures, adding mechanical and thermal stresses on the casing. Deep gas wells in the Gulf Coast can approach reservoir pressures in the tens of thousands of psi and temperatures in the 300–400 °F range. Casing must have sufficient burst, collapse, and tensile ratings to withstand these loads (often needing higher grade or thicker-walled pipe). However, higher-strength grades (like P110, Q125) typically achieve their strength via higher hardness, which is detrimental for SSC resistance. This creates a materials engineering dilemma: the casing must be strong enough for HPHT loads yet soft enough (low hardness) to be SSC-resistant. Temperature also affects material behavior; at elevated temperatures, steel yield strength reduces and corrosion rates can change. Some CRA materials (e.g. duplex stainless) have upper temperature limits for sour service due to chloride stress cracking concerns. The HPHT nature of the well may thus push the selection toward specialized grades or thermal treatments that retain strength at temperature while meeting sour service criteria.
Zonal Isolation and Fluids: Sour HPHT wells demand high reliability in cementing and completion as well. H₂S can permeate through micro-annuli or poorly bonded cement, so casing materials and cements must maintain a seal under corrosive conditions. CO₂ and H₂S can degrade oilwell cements over time (CO₂ forms carbonates, H₂S can form sulfides/sulfates), potentially leading to loss of zonal isolation. Class H cements with anti-corrosion additives are often chosen for better resistance in such environments. The casing material should also be compatible with completion fluids: for example, high-chloride brines or packer fluids can cause pitting in 13Cr or duplex steels if not carefully inhibited. Completion operations like acid stimulations need consideration too – acidizing a formation with HCl/HF requires that production casing (or liners) either be protected by an inhibitor or made of CRA to avoid rapid acid attack. In sum, the sour HPHT environment imposes multifaceted challenges – SSC/HIC, CO₂ corrosion, extreme mechanical stress, and chemical compatibility – all of which inform the casing grade selection.
Engineering Criteria and Standards for Material Selection
API 5CT Casing Grades: The starting point is API Specification 5CT, which defines standard OCTG casing grades with different yield strengths and chemical requirements. API grades commonly used in deep wells include L80, C95, T95, P110, Q125, among others, each with specified minimum yield and tensile strengths. For sour service, API 5CT provides guidance (in Annex H / Section on SSC testing) for certain grades that are sour-service qualified (often referred to informally as “NACE grades”). Notably, L80 Type 1, C90, C95, T95, and even a category of C110 are produced with tighter chemistry (lower sulfur/phosphorus) and are subjected to SSC testing per NACE TM0177. These grades have controlled hardness (typically ≤22 HRC for L80 and ≈ 25 HRC for 95 ksi grades) to improve SSC resistance. In contrast, high-strength grades like P110 and Q125, while offering high load capacity, are not recommended for use in H₂S environments unless specially qualified, because steel “becomes more susceptible to sulfide stress cracking the higher the yield strength”. API 5CT thus essentially limits Region 3 sour service applications to the lower-strength grades (up to 95–110 ksi yield). Engineers must match the API grade’s strength to well design loads, then check if that grade is acceptable for the H₂S level. Often a heavier wall or additional casing string may be needed if a lower-strength grade is used to handle pressures.
NACE MR0175 / ISO 15156: This international standard is the bible for materials in oilfield H₂S service, focusing on preventing SSC and related cracking. NACE MR0175/ISO 15156-2 (Part 2) addresses carbon and low-alloy steels, setting environmental limits and qualification requirements. It essentially requires that if H₂S ≥0.05 psi, you must use SSC-resistant materials or prove resistance. The standard imposes a maximum hardness of 22 HRC on most steels for sour service, and often requires qualifying susceptible grades via laboratory SSC tests (e.g. NACE TM0177 Method A) in simulated sour brines. For OCTG, NACE references API 5CT “sour service” casing grades that have been tested. It’s the operator’s responsibility to ensure a given casing grade is suitable for the specific H₂S partial pressure, pH, chloride, and temperature of the well. For example, L80 and T95 are acceptable in “Region 3” sour environments (high severity) if hardness and tests are in order, whereas 13Cr stainless may only be used in “Region 1” or mild sour (e.g. H₂S <1.5 psi, pH ≥3.5). ISO 15156-3 (Part 3) covers CRAs and defines which stainless steels, duplex, and nickel alloys can be used at given H₂S levels, temperatures, and chlorides. Following NACE MR0175 ensures we address SSC, HIC, SOHIC (stress-oriented HIC), and SCC in the material selection. In practice, most casing suppliers certify certain grades as “NACE compliant” for sour service by performing the requisite tests and controls.
API Technical Reports and RP: In addition to API 5CT, several API publications guide casing selection for extreme wells. API TR 5C3 provides formulas for casing performance properties (burst, collapse, tension) which are vital for designing a deep well casing string. HPHT wells often require casing with enhanced collapse resistance (due to high external pressures from dense mud at 27,000 ft). Manufacturers offer high-collapse versions of grades (achieved via special heat treatments and tolerances) that still meet sour service criteria. Engineers should check collapse ratings (per API TR 5C3/ISO 10400) for the selected grade and weight, especially for deep intermediate and production casing. For HPHT environments, API TR 1PER15K-1 and related documents classify HPHT equipment and materials – these emphasize using validated materials for >15 ksi, >350°F service. They don’t override NACE, but they underscore that material verification and quality is crucial at these extremes. It’s also wise to consult API Recommended Practices (RPs) like API RP 5C1 and 5C5 for inspection/testing, and any relevant API technical bulletins on sour service failures. The industry’s collective experience is often captured in case-study papers and guidelines (e.g. NACE papers, SPE/IADC conference proceedings) which can provide insight on selecting casing for similar deep sour wells.
Casing Material Options for Sour Service
Selecting the optimal casing material involves choosing between carbon steel grades (with proper sour service modifications) and corrosion-resistant alloys (CRAs), or a combination thereof. Below we compare common options and their suitability:
L80 Type 1 (Carbon Steel): L80 is a 80 ksi yield, quenched-and-tempered carbon steel. Type 1 L80 has no deliberate alloying for corrosion resistance (unlike 13Cr) but is a popular choice for sour wells because it’s relatively low in strength (easier to keep below 22 HRC hardness) yet strong enough for moderate depths. It is fully SSC-resistant in Region 3 environments when produced to NACE specs. Its pros are cost-effectiveness and wide availability – it’s a basic API grade, thus numerous mills stock it. However, in a 27,000-ft HPHT well, pure L80 may lack sufficient strength for deep casing strings; its 80 ksi yield might require thick walls that reduce internal diameter or a multi-string design to handle the pressure. Also, L80’s CO₂ corrosion resistance is only as good as any carbon steel – it might need chemical inhibition if exposed to produced fluids with CO₂. Still, L80 is often used in intermediate sour zones and as production casing in many sour gas wells where pressures allow.
L80 Type 13Cr (Martensitic Stainless): This is a 13% chromium stainless steel tempered to about 80 ksi yield (technically a subclass of L80). L80-13Cr is a popular CRA for “sweet” CO₂ environments and mildly sour wells because the chromium provides a protective oxide layer greatly reducing CO₂ corrosion. In wells with high CO₂ and low H₂S, 13Cr tubing or casing can drastically extend life compared to carbon steel. It’s often considered when CO₂ corrosion is the main threat. However, caution: 13Cr is susceptible to SSC if significant H₂S is present. NACE MR0175 imposes an environmental limit (~1.5 psi H₂S, pH ≥3.5) for 13Cr and similar martensitic steels. Beyond those mild-sour limits, 13Cr can crack just like high-strength carbon steel. Additionally, 13Cr is vulnerable to chloride stress corrosion cracking at high temperatures, and pitting in the presence of chlorides if oxygen enters the system. In a Gulf Coast well with high H₂S, L80-13Cr would not be suitable for primary casing exposed to production fluids – it might be used in upper sections where exposure to sour fluids is minimal, or perhaps as a liner in a CO₂-heavy but low-H₂S zone. Cost-wise, 13Cr is more expensive than L80 carbon steel (due to alloying and more complex heat treatment) but cheaper than higher-end CRAs. It often provides a good compromise for moderate depth, CO₂-rich, low-H₂S scenarios.
C95 / T95 (Sour Service 95 ksi Carbon Steel): These grades have 95 ksi minimum yield, offering more strength than L80 while maintaining sour service capability. API distinguishes T95 as a tubing grade with two types (Type 1 and 2 differing in chemistry) and requires SSC testing; C95 is a casing-grade that in some specs doesn’t mandate SSC tests (sometimes called R95 for “Reference 95” with no NACE). In practice, however, many mills produce 95 ksi casings to meet sour service requirements, whether labeled C95 or T95. The advantage of 95 ksi grade is higher load capacity – critical for deep wells – while still being SSC-resistant in severe sour (Region 3) if properly processed. T95 has max hardness around 25 HRC and must pass NACE TM0177 SSC tests, making it suitable for severe sour service. These grades are commonly used for deep sour wells’ production casing. For our 27,000-ft well, a string of T95 casing could handle higher pressure than L80 with the same wall thickness. The trade-off is that as strength rises, toughness and SSC margin shrink – the material is closer to the threshold of cracking, so quality control is paramount. Also, 95 ksi sour casing costs a bit more than L80 (due to extra testing and tighter chemistry). It’s still far cheaper than exotic CRAs and usually available from multiple suppliers. In many cases, T95 is the highest API grade reliably usable in high-H₂S environments
.
110–125 ksi “Sour” Grades (C110, proprietary 110–125): To meet very high pressure requirements in sour wells, some manufacturers offer proprietary grades in the 110–125 ksi range that are specially engineered for sour service. API C110 is one such grade (110 ksi yield) intended for sour use, with chemistry and hardness controls similar to T95. However, standard C110 is actually only qualified for moderate sour (NACE Region 2) in many cases – one source notes that despite testing at 1 bar H₂S, C110’s acceptable domain is limited to Region 2 (lower severity). This means pure C110 may not be suitable if our well’s H₂S is extremely high or pH very low (Region 3). To bridge the gap, companies like Vallourec, Tenaris, TMK, etc., developed proprietary high-strength sour grades: e.g. VM 110 SSC (Vallourec), TN 110SS (Tenaris), or TMK’s P110 MS series, which tweak alloying and tempering to improve SSC resistance. These have been tested to certain H₂S limits and stresses. For instance, Vallourec’s VM 125 SS (125 ksi) has been qualified for slightly sour conditions (0.03 bar H₂S at pH 3.5) for deep HPHT use, and they report deploying 125 ksi sour casing in Gulf of Mexico HPHT projects. In general, pushing strength this high for sour service comes with performance trade-offs: the alloys require very clean steel (low impurities), precise heat treatment, and often have lower toughness. They also may be limited in the sour severity they can tolerate (often “mild sour” only). In our exploratory well scenario, one might use a proprietary 110–125 ksi sour grade if absolutely needed for load reasons – for example, a deep intermediate casing where H₂S exposure might be lower (if isolated by another string), or as a production casing if the H₂S level is at least moderate and well-studied. Otherwise, beyond ~95 ksi the safer choice in truly sour (high H₂S) conditions tends to shift to CRAs.
Corrosion-Resistant Alloys (CRA): These include high-chromium stainless steels, duplex stainless steels, and nickel-based alloys that provide superior resistance to H₂S and CO₂. They are considered when carbon steel cannot reliably handle the environment or design life. Common CRA options for sour wells:
Super 13Cr (13Cr110): An improved martensitic stainless with ~110 ksi strength and slightly more chrome/moly than standard 13Cr, giving better SSC resistance and pitting resistance. Super 13Cr can handle moderate sour (perhaps a few psi H₂S) and higher temperatures than 13Cr, but it’s still not for “unlimited H₂S.” It’s mainly used for high-CO₂ wells with some H₂S.
Duplex Stainless (22Cr, 25Cr): Duplex alloys (with mixed austenitic-ferritic microstructure) like 22Cr and 25Cr offer high strength (typically 110–125 ksi) and excellent CO₂ and chloride corrosion resistance. 25Cr super duplex is even NACE-qualified for quite sour conditions (e.g. 3 psi H₂S at up to 232 °C for certain grades). They have been used as production tubing and liners in extremely sour gas wells (e.g. Middle East fields with 20–30% H₂S). Duplex casings could be considered if H₂S is very high and a single-string completion is needed to directly contain production fluid. However, duplex can be prone to sulfide stress cracking if not properly heat-treated, and care must be taken with welding. Also, duplex’s cost is several times that of carbon steel.
Nickel-Based Alloys: These include Incoloys and Inconels (e.g. 825, 925, 718, C276). They have near-immunity to H₂S cracking up to very high pressures and temperatures. For example, alloy 718 (a high-strength nickel alloy) can reach 140 ksi yield and is used in subsurface safety valves and packers in the harshest sour wells. In tubular form, Alloys like 925 or G3/G50 (low alloy nickel-chrome) and 825 (Ni-30%Fe-22%Cr) have seen use as tubing or liners in severe sour/high-CO₂ wells where even super duplex might suffer. These materials are extremely costly (dozens of times more expensive than carbon steel) and are usually only justified for short sections like production tubing, liners, or cladding, rather than full-length large-diameter casings.
Clad or Lined Pipe: A cost-mitigating option is to use composite strings – e.g. carbon steel pipe internally clad with a thin layer of CRA (metallurgically bonded) or with a plastic/composite liner. Clad OCTG gives you the strength of carbon steel with the corrosion resistance of CRA on the bore. While more common in pipelines, clad casing or tubing is also used downhole. For instance, a P110 casing could be internally clad with Alloy 825 to withstand sour production. This can be less expensive than solid CRA but is still an advanced solution, with challenges in cladding weld quality and ensuring no holidays in the CRA layer.
In summary, carbon steel grades (L80, C95, T95) are workhorse choices for sour wells, provided the H₂S levels are within their proven capacity. For the most severe sour/HPHT conditions, CRA materials may be needed for production tubing or flow-wetted parts, with carbon steel used in outer casings that see buffered environments (e.g. cemented annulus, inhibited packer fluids). Sometimes a combination is used: for example, a T95 production casing for strength, paired with a CRA tubing that actually contacts the sour flow, thereby protecting the casing from produced fluids.
SSC, HIC, and CO₂ Corrosion Considerations
Sulfide Stress Cracking (SSC): Preventing SSC is paramount in high-H₂S wells. Engineering mitigations include specifying a maximum hardness (typically 22 HRC for most steels) and requiring post-weld heat treatment of any welds to reduce hardness in heat-affected zones. OCTG casing is usually seamless or ERW, so welds aren’t a factor except at connections – any weld repairs on casing or attachment welds (like on accessories) must also adhere to NACE hardness limits. Material selection wise, SSC drives us to use quenched & tempered low-alloy steels with tempered martensite microstructure, which combine strength with toughness and lower hardness. Impurity control is also important: high sulfur inclusions can act as initiation sites for hydrogen blisters. Sour-service casing steels therefore have tighter S and P specs and often calcium treatment to modify inclusions. Vendors typically certify casing grades by actual SSC lab testing – for example, specimens of C95 or T95 are tested per NACE TM0177 in a test solution (acidified brine with H₂S) under 80% of yield stress for 720 hours. Passing such tests gives confidence the material can tolerate field stresses without cracking. It is worth noting that SSC susceptibility generally increases as temperature decreases from high to moderate – thus a material that is safe at 250°F downhole might crack at 80°F near the wellhead. We design for the worst-case, including cooldown scenarios. In practice, proper grade selection (as discussed above) along with maintaining compressive residual stresses on pipe inner surfaces (sometimes done via shot-peening or autofrettage in extreme cases) and controlling operating conditions (avoiding sudden pressure drops that promote rapid hydrogen entry) are measures to combat SSC.
Hydrogen-Induced Cracking (HIC): HIC is a form of damage where hydrogen (from H₂S corrosion) diffuses into the steel and collects at inclusions or voids, forming internal blisters and laminations without externally applied stress. This is more of a concern for plate materials (e.g. sour service line pipe and pressure vessels) but can occur in casing if the steel has a high level of sulfur inclusions and is exposed to standing sour fluids. HIC-resistant casing would be made from very clean steels (low sulfur, small grain inclusions). While API 5CT doesn’t specifically mandate HIC tests for casing, some operators do require HIC testing per NACE TM0284 for critical wells. Generally, the same practices that yield SSC-resistant steel (low impurities, calcium treatment, etc.) also improve HIC resistance. For a deep well, HIC could be a concern if sections of casing are continuously immersed in sour brine (e.g. water-filled segment with H₂S). If HIC is expected, one might upgrade to CRA or consider lining the casing. The presence of CO₂ can actually mitigate HIC to a degree by promoting a protective carbonate scale; however, CO₂ also lowers pH which can increase hydrogen flux. It’s a complex balance, and material selection errs on the side of caution by using proven “sour service” grades with good HIC track records.
CO₂ Corrosion Control: Unlike SSC, which is a sudden fracture risk, CO₂ corrosion is a gradual wall-thickness loss issue (though it can pit). Designing for CO₂ involves estimating corrosion rates and deciding if corrosion allowance or mitigation is needed. If using carbon steel for production casing, engineers may account for a certain corrosion allowance (extra thickness) and deploy corrosion inhibition in the produced fluids. Continuous injection of corrosion inhibitor chemicals can protect carbon steel casing/tubing in CO₂/H₂S service, but reliability of inhibition must be high to avoid upsets. When CO₂ partial pressure or temperatures are very high, the safer option is a CRA. For example, 13Cr or 22Cr duplex casing might be justified if predicted CO₂ corrosion rates on L80/T95 are too high to manage. We also consider that temperature greatly influences CO₂ corrosion – peak attack often occurs in the 120–250°F range; above that, iron carbonate scales may form more stably and reduce corrosion, and at very high temp the kinetics slow. In our Gulf Coast well, if bottomhole temps ~350°F with CO₂ present, some protective scaling could occur, but near the cooler top of the well CO₂ could be quite aggressive until fluids cool below water dewpoint. Thus, an integrated approach is taken: material selection plus chemical treatment plus possibly coatings (e.g. internal epoxy coating in less critical casings) to handle CO₂.
Cost and Supply Chain Considerations
When evaluating casing materials, cost and availability play a significant role, especially for exploratory wells where budget and timing are key. Carbon steel casing grades are generally the most cost-effective option, as they leverage mass production and established supply chains. L80, P110, etc., are commodity grades – price per ton is relatively low, and local suppliers in the Gulf Coast usually stock these or can source them quickly. Upgrading to CRA or specialty sour grades can multiply costs. For instance, a 13Cr or duplex stainless casing can cost several times more per foot than an L80 joint. Nickel-based alloy tubulars are exponentially more expensive. Moreover, CRA connections often require premium threading (VAM, TenarisHydril, etc.) which adds cost.
Supply chain logistics in the Gulf Coast need consideration. High-performance alloys may have long lead times since only a few mills globally produce them in oilfield tubular form. An operator might wait 6–12+ months for delivery of large-diameter CRA casings. Even for sour-service carbon grades, the surge in demand for alloy grades worldwide can impact lead times. A report by Fastmarkets noted that increased global drilling in sour fields has put pressure on traditional OCTG supply chains, with mills extending lead times for alloy grades and even established buyers in regions like the Gulf of Mexico facing delays. Thus, early procurement and possibly strategic inventory are needed if exotic grades are chosen. The Gulf Coast region does benefit from proximity to OCTG manufacturers (some U.S. mills and heat treaters can turn around sour service casing relatively quickly) but for CRAs, one might rely on imports from Europe or Asia. Sourcing challenges also include quality consistency – for critical sour service, operators often stick to known manufacturers with proven QA/QC, even if cheaper options exist, to avoid counterfeit or subpar steel that could fail. This can narrow the supplier pool and give certain mills significant lead times.
In terms of cost trade-offs, there is always a tension between up-front material cost and long-term performance. Using carbon steel casing with inhibition is cheaper initially, but carries risk of future intervention or failures if corrosion monitoring and inhibition falter. Using a CRA is capital-intensive, but potentially secures the well’s integrity for its full life with less maintenance. A cost-benefit analysis might consider the consequences of a casing failure (which in a sour well could be disastrous for safety and environment) versus the added completion cost of CRA. For an exploratory well, sometimes operators opt for a conservative (but cheaper) approach – e.g. run sour-service carbon steel casing, but design the completion so that production tubing (which is retrievable) is CRA. That way, the CRA tubing protects the casing from direct exposure; if the tubing fails, it can be replaced, whereas the primary casing is not as exposed. This approach can balance cost and risk.
Finally, it’s worth noting the market volatility: alloy prices (for chromium, nickel, molybdenum) can fluctuate, impacting CRA costs. In recent years, supply chain constraints and high demand have driven up prices for 13Cr and above materials. Fabrication of CRA pipes is also more complex – slower rolling and heat treating – which adds to cost. These practical factors often make engineers lean towards “fit for purpose” selection: use the cheapest material that meets the technical requirements with acceptable risk.
Completion and Cementing Compatibility
Selecting the casing grade is not done in isolation; it affects and is affected by the overall well completion plan:
Cementing: The metallurgy can influence cementing practices. With carbon steel casing, standard Class H cement with anti-gas-migration additives is common. In a sour well, adding sulfide scavengers to the cement slurry can protect the casing from H₂S during the cement cure. If using a CRA casing, one must ensure the cement (or spacers) do not contain chemicals that could attack the CRA’s passive film. For example, certain oxygen-rich latex additives might cause a flash of corrosion on 13Cr if not handled. Cement bonding to different metals is usually not a problem (steel of any kind bonds similarly), but thermal expansion differences could be if a CRA has a different expansion coefficient – however, steels and CRAs are generally in a similar range, so this is minor. More important is gas-tight cement: H₂S can percolate through microcracks over time, so premium cements or resins may be used to ensure an enduring seal. If a dual-string design is used (e.g. a CRA liner inside a carbon steel casing), cementing that liner in place might require special techniques to avoid galvanic couples (see below).
Galvanic Considerations: If different casing materials are used in the well (for instance, a 13Cr tubular in contact with a carbon steel casing or coupling), galvanic corrosion could occur in the presence of a conductive fluid. Typically, the more anodic metal (carbon steel) would corrode preferentially, protecting the noble (CRA) metal. In downhole conditions, galvanic effects are often limited by the low oxygen and the fact that both metals might be coated with films or cement. But one should consider using insulating sleeves or connections if a long CRA section is directly coupled to carbon steel and exposed to brine. This is especially relevant for tie-back liners or work strings – completion engineers sometimes deploy bimetallic transition joints or isolators to break the galvanic path between production tubing (CRA) and casing (carbon steel).
Packer Fluids: The fluid left in the casing-tubing annulus (packer fluid) is critical to corrosion control. For a sour well, this fluid is often a brine with corrosion inhibitor and oxygen scavenger. If the casing is carbon steel, the packer fluid must be aggressively treated to avoid corroding the casing from the outside. If the casing is CRA, one must ensure the packer fluid is compatible with it – for example, high-density ZnBr₂ brines are known to cause stress corrosion cracking in 13Cr and duplex steels at elevated temperatures. So, if a heavy completion brine is needed, a corrosion inhibitor specifically formulated for the CRA may be required, or the fluid may need to be swapped out to something like CaCl₂/CaBr₂ blend which is less harsh. This is a good example of how completion strategy (choice of packer fluid) and material selection go hand in hand.
Production Operations: Using a high-alloy casing can offer more flexibility in production (e.g. allowing acid stimulation or high-rate gas flow with less worry about erosion/corrosion on the pipe). However, high alloy casings require different handling – for instance, 13Cr is less forgiving of rough handling; galling can occur on threads, so dope selection and running practices are adjusted. During perforating, one must consider that stainless steels have different shot penetration characteristics than carbon steel (not usually a big issue, but worth noting for perforation design).
Well Intervention and Repair: If the well ever needs a workover, knowing the casing material is vital. For example, if milling or cut-and-pull is required, CRA materials can be harder on tools. Likewise, if a liner hanger or packer is set in CRA casing, the slip design may need to account for the casing’s hardness so it can bite properly. Completion engineers will plan equipment (packers, hangers, seals) that are compatible with the casing metallurgy – elastomer seal selection particularly must account for any sour fluids permeating the materials.
In summary, the chosen casing grade must integrate with the cementing plan (to ensure long-term zonal isolation in sour conditions) and with completion fluids and hardware (to avoid corrosion or material conflicts). Often a cross-disciplinary review (materials, drilling, completion, production teams) is done for HPHT sour wells to ensure the casing material will perform from drilling all the way through production and possible abandonment.
Conclusion
Designing casing for a 27,000-ft sour gas well in the Gulf Coast requires a meticulous balance of strength, toughness, and corrosion resistance. Engineering criteria grounded in standards like API 5CT and NACE MR0175/ISO 15156 guide us toward materials that can withstand both the extreme pressures/temperatures of deep drilling and the chemically aggressive nature of H₂S/CO₂. In practice, this often means favoring sour-service carbon steel grades (L80, C95, T95) for their proven SSC resistance at moderate strength, while judiciously deploying CRAs (13Cr, duplex, nickel alloys) for the most corrosion-prone sections or when mechanical demands exceed what carbon steel can safely handle. We compared these options: carbon steels are economical and readily available but need careful control of hardness and ongoing corrosion mitigation, whereas CRAs offer inherent corrosion protection at a steep cost and with supply considerations. The decision also hinges on how the well will be completed – for instance, one strategy is using a carbon steel casing with a CRA tubing to shield it from sour production fluids, which can be cost-effective.
Ultimately, materials selection for sour HPHT wells is a risk management exercise. The goal is to ensure well integrity over the life of the well, preventing failures that could lead to hazardous sour gas releases. This means erring on the side of safety with regard to SSC – using only qualified grades and possibly derating high-strength steel usage in sour sections. It also means engaging casing suppliers early to secure the needed metallurgy in time. For our deep Gulf Coast well, a likely casing program might use T95 or proprietary 110SS for the production string (if the H₂S level is within its limits) and perhaps a 13Cr or duplex liner if a particularly corrosive zone is encountered, all cemented with an H₂S-resistant slurry. This approach leverages the strengths of each material. In all cases, adherence to industry standards and best practices is crucial – these wells push materials to their limits, so the lessons captured in API TRs and NACE directives are invaluable in guiding safe casing design.
By thoughtfully considering SSC, HIC, CO₂ corrosion, and the interplay of material with the well design, drilling engineers and well integrity specialists can confidently select a casing grade (or combination of grades) that will withstand the sour HPHT environment. The result is a robust casing design that protects both the asset and the people and environment around it, ensuring successful exploration and production of these challenging deep sour gas reserves.
References:
API Specification 5CT – Specification for Casing and Tubing, American Petroleum Institute. (for steel grade definitions and requirements)
NACE MR0175 / ISO 15156 – Petroleum and natural gas industries — Materials for use in H₂S-containing environments in oil and gas production. (for sour service qualification of metals)
Sovonex, “API Casing Grades – An Overview.” (summary of casing grades and their sour service applicability)
Vallourec, “Sour Service Solutions.” (manufacturer data on sour service grades and
Emerson (Fisher) Technical Paper, Sulfide Stress Cracking – NACE MR0175/ISO 15156 (discussion of NACE limits for various materials)
Element Materials Technology, “NACE MR0175/ISO15156 Sour Service Application.” (overview of SSC, HIC test requirements)
Fastmarkets, “Explosion in chromium and nickel OCTG demand” (notes on supply chain impacts for CRA OCTG)
Permanent Steel, “Carbon Steel vs Stainless Steel Casing.” (comparative discussion of properties and cost)
Schlumberger Oilfield Review, “Defining HPHT Wells.” (API HPHT criteria and challenges)
TMK IPSCO, Sour Service OCTG Brochure (product data showing NACE Regions and grade capabilities)
Comments